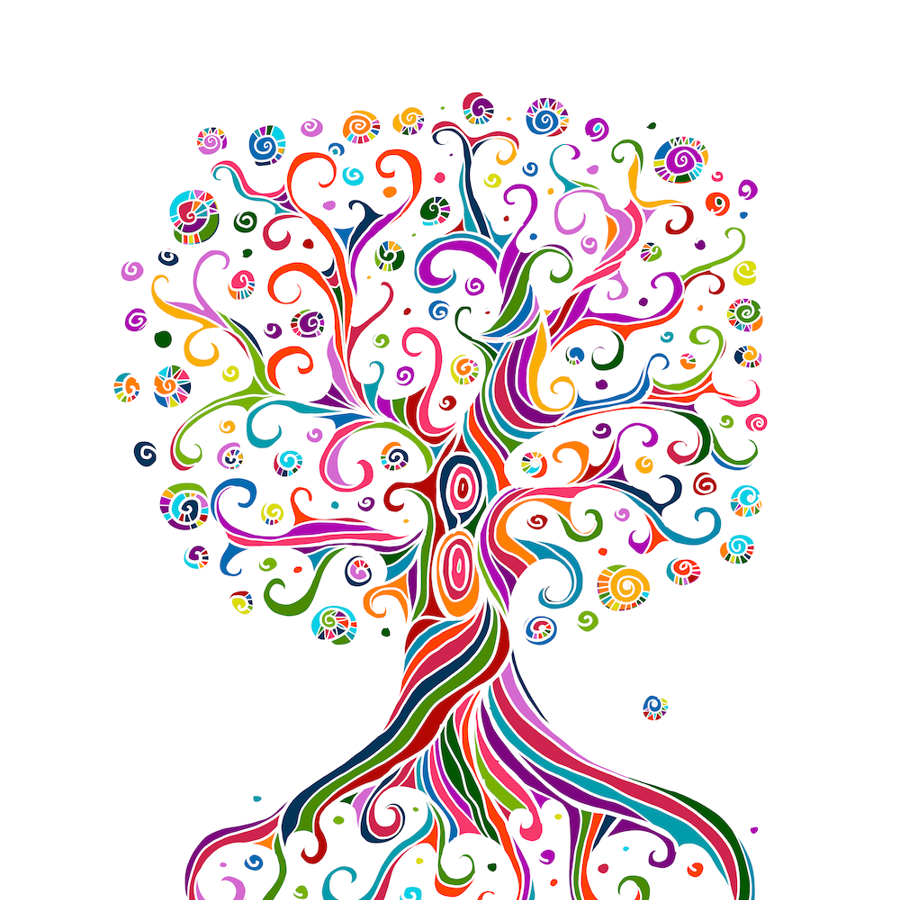
For how long can DNA transfer from generation to generation?
June 26, 2024
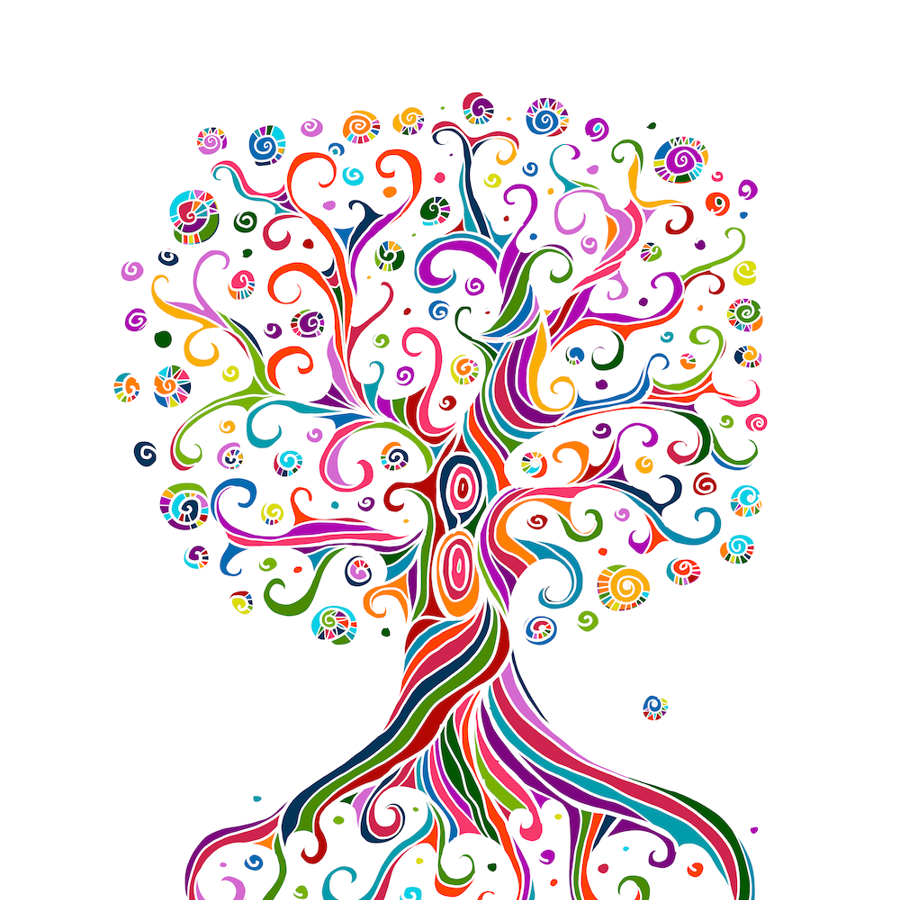
- Related Topics:
- Ancestry,
- Y chromosome,
- Mitochondria,
- Evolution
A curious adult from Ethiopia asks:
"For how long can DNA transfer from generation to generation? Is it possible that I can have similar genetic material like my ancestors?"
You thought your grandma’s spaghetti recipe was ancient? The recipes in our DNA trace back millions of years! But before we dive into it, let’s first understand how DNA works.
DNA is like a cookbook
You can think of DNA as a cookbook with all the recipes needed to make a person. Each page of the book contains a single gene, which is like a recipe for a specific protein. Our cells are constantly reading the DNA cookbook to make a whole banquet of proteins that keeps us alive and healthy!
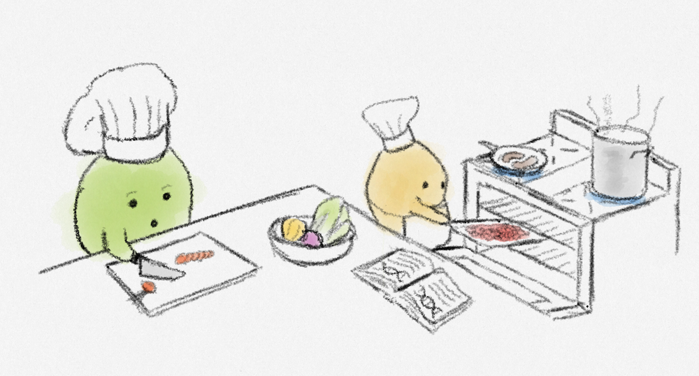
Passing along our DNA
So how are DNA cookbooks passed down from generation to generation? Well for starters, we actually have two copies of the cookbook – one inherited from each parent. So, for most proteins, we actually have two copies of each recipe, which may be slightly different.
Our cells typically use both copies of each recipe, producing both versions of the protein. However, we can only pass down one copy to our children. To do this, we blend together our two cookbooks into a single new book, with only one copy of each recipe. The child inherits this new book plus a second new book from their other parent, so they end up with two copies of each recipe.
Since one cookbook comes from each parent, our DNA is roughly 50% from mom and 50% from dad. And each parent’s cookbook is a mixture of their parents’ cookbooks, so we owe 25% of our recipes to each grandparent, 12.5% to each great grandparent, and so on. By the time we go ten generations back, each ancestor contributes less than 0.1% of our DNA cookbook.
This percentage is so small because the farther we go back in the family tree, the more and more ancestors we have. Our DNA cookbooks are a melting pot of every cookbook that came before us!
Our ancestors’ cookbooks weren’t handed down to only us. Our relatives also inherited many of the same recipes!
Tracking relatives with DNA
If you are related to someone, you both inherited DNA from a common ancestor. For instance, your common ancestor with your cousin is your shared grandparents.
Since your grandparents handed down their recipes to both you and your cousin, you both inherit many identical recipes. In contrast, an unrelated person would also have recipes for these proteins, but their recipes might have slight differences. These differences indicate that the recipes weren’t inherited from a recent common ancestor.
So, we can determine if you are related to someone by scanning your cookbooks for identical recipes. However, detecting distant relatives is more challenging, since more and more recipe shuffling occurs over generations. According to 23andme, you have a 90% chance of detecting a third cousin and a less than 5% chance of detecting a sixth cousin. It quickly becomes hard to trace ancestry once we are separated by this many generations!
Luckily, there are two types of DNA that don’t participate in cookbook shuffling. We can use these to trace specific lines of ancestry back much farther!
The first type is mitochondrial DNA, which exists in tiny energy-producing factories (called mitochondria) inside of cells. Consider this DNA as an extra booklet of recipes. We inherit our mitochondrial DNA directly from our mother’s egg, who inherited it from her mother’s egg, and so on.
The other type is Y chromosome DNA. This DNA is present only in biological males and is passed directly from fathers to their sons. Your father received his Y chromosome DNA from his father, who received it from his father, and so forth.
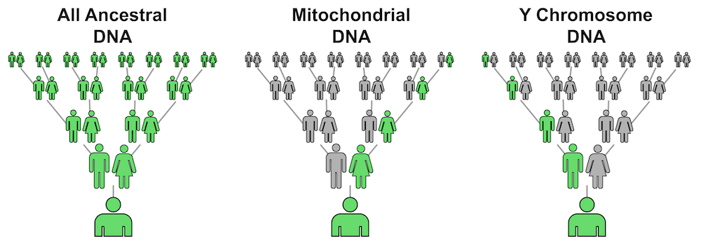
Mitochondrial and Y chromosome DNA only track a single maternal or paternal line of ancestors, as opposed to all of our ancestors. This means that we know the exact people in our family tree who passed these recipes down to us!
It also means we can track them back much farther than the rest of our DNA. Scientists estimate that mitochondrial DNA of every living person today comes from a single female ancestor roughly 150,000 years ago1. Similarly, all Y chromosomes can be traced back to a single male ancestor who lived 200,000-300,000 years ago2. It’s important to note that these were not the first humans; they’re just the first humans whose mitochondrial and Y chromosome DNA wasn’t lost to time.
Tracing even further back
We can actually trace DNA much, much further back in time. For instance, maybe you’ve heard that humans are about 96% similar to chimpanzees.
This is because we share ancestry with chimps – at some point we shared the same set of recipes.
But our cookbooks have changed with time. Whenever DNA is passed along to the next generation, there are chances to make some mistakes, or mutations. In our cookbook analogy, this would be like making typos in the recipes you pass to your child.
These typos can change the recipe. Over long periods of time, the recipes can change significantly. But we can often still tell that they have the same origins.
For example, maybe one species has a recipe for a good crusty bread, while another has a recipe for a soft dinner roll. We can tell those are related, even if they aren’t exactly the same.
And while many parts of our DNA are very different from other species, certain portions of DNA are so essential that we find them all across the evolutionary tree. These portions are said to be evolutionarily conserved, meaning they don’t change much with evolution.
Evolutionarily conserved DNA is like a foundational recipe. If it gets messed up, it’s unlikely that the organism will survive to pass along the DNA. As a result, this DNA doesn’t tend to change much between generations.
One example of an evolutionarily conserved gene is Pax6. It’s an essential gene that makes a protein needed to form eyes. If mice are missing their Pax6 recipes, their eyes don’t properly form lenses3. Pax6 certainly is a recipe we don’t want to mess up!
It turns out many other species use Pax6. Fruit flies also use the Pax6 gene to form their compound eyes. Even though a human and a fly eye look nothing alike, the fruit fly version of Pax6 is 94% similar to the human version4.
Even more remarkably, mouse and fly versions of Pax6 may be able to substitute for one another. If you insert the mouse version of Pax6 into a fly, it will cause a compound eye to grow wherever the gene is placed5! (You can actually get flies to grow eyes on their legs this way!) Fly cells don’t care that the recipe is from a mouse – the recipe is close enough.
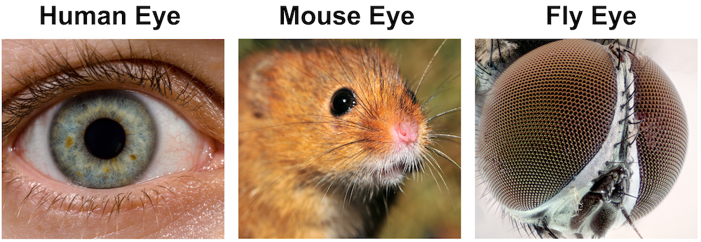
Flies, mice, and humans didn’t stumble upon the perfect, nearly-identical Pax6 recipe by chance. We all inherited this recipe from a common ancestor hundreds of millions of years ago. We think this ancestor was a worm-like creature that also used Pax6 to form eyes6. This worm’s recipe was handed down from generation to generation as new species split off. As a result, humans, mice, flies, and countless other species have inherited the Pax6 gene. Talk about a family recipe!
Today, humans and flies could not look more different. Despite this, we both still hang on to the same Pax6 recipe after millions of years of evolution. And Pax6 is just one example – there are many other genes that haven’t changed much as species evolve.
In conclusion
Our DNA is more than just a cookbook; it is a chronicle of the millions of years of culinary refinement that has kept us alive. Each recipe tells a story of how our ancestors survived, whether they be modern humans or ancient worms. As we come to better understand our own DNA, we continue to uncover more about those who came (and cooked) before us.
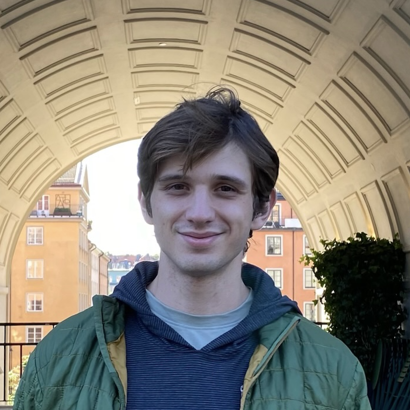
Author: Raleigh Slyman
When this answer was published in 2024, Raleigh was a Ph.D. candidate in the Department of Mechanical Engineering, studying human embryo organization in Ovijit Chaudhuri’s laboratory. He wrote this answer while participating in the Stanford at The Tech program.