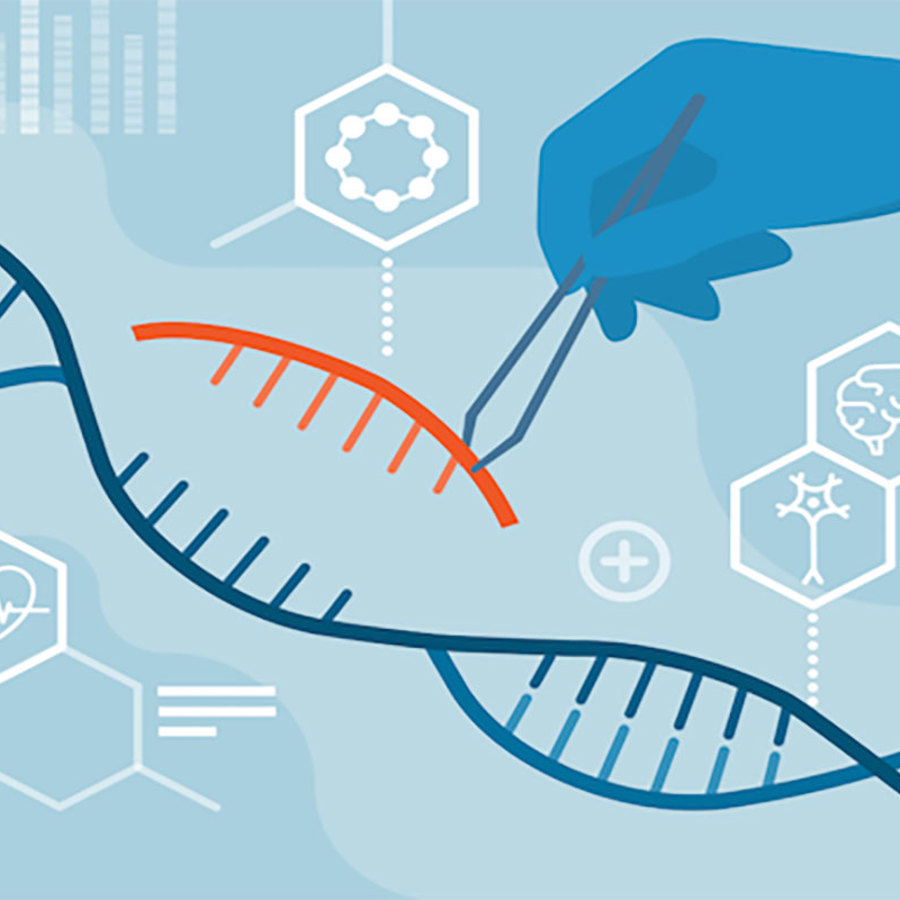
What is CRISPR and how does it work?
May 4, 2016
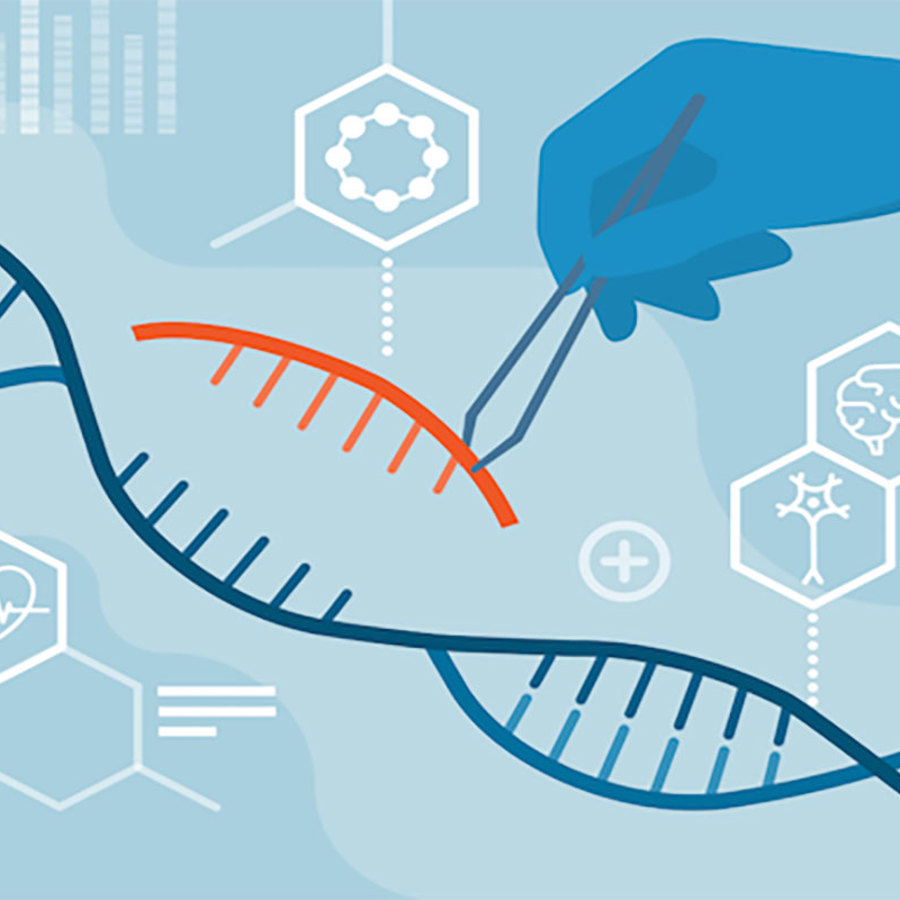
- Related Topics:
- Gene therapy,
- Genetic engineering,
- CRISPR,
- RNA,
- Biotechnology
A curious adult from California asks:
“What is CRISPR and why is it so great?”
CRISPR is a game changing DNA editing tool that has already revolutionized biology research and may be set to do the same for medicine and patient treatment. It is truly a game changer.
What makes it such a breakthrough is how easy it is to create the edit in a very specific spot in a cell’s DNA (its genome). This is quite a feat given that the right spot is somewhere in the six feet of 6 billion microscopic DNA letters scrunched together inside of a microscopic cell.
For several decades scientists have created tools that let them edit the DNA in some way, but redirecting them to a specific DNA target is much harder than with CRISPR. CRISPR is the first system where getting to the right spot is almost trivial.
Think about it like a map on a phone app. With CRISPR, you basically enter an address into a mapping app and bam, you get to the right place. With an old map, you couldn’t do that.
Once you get past targeting, all of these tools edit DNA in essentially the same way. They all cut the DNA at some spot, and then let the cell deal with that cut — as in, sew itself back up in whatever way it wants.
Sometimes the cell messes up the gene as it tries to sew itself back up. But occasionally, if you add a piece of DNA to the cell that has the specific edit you want to make, the gene gets edited in the right way. It is that word “occasionally” that makes CRISPR a much more powerful tool for basic science research rather than curing genetic diseases directly.
See, in basic biology research if just a few cells get the DNA change you want, that is OK. You can separate them from the other cells and use just them. After growing these altered cells for a while, you’ll end up with plenty of cells with the changed DNA.
You can even do this with a whole animal. You might use CRISPR on a bunch of fertilized eggs and only use the ones that got the change. Or if only some of the cells in the animal got the edited DNA, you can mate the animal and pick the offspring that have the change in every cell.
This approach won’t work with most medical treatments though — changing the DNA in just a few cells in patients may not affect their health in any meaningful way. For example, fixing the DNA mutation that leads to cystic fibrosis in less than 1% of lung cells is simply not going to help a lot. (Diseases of the blood may be an exception because they can be removed easily and added back.)
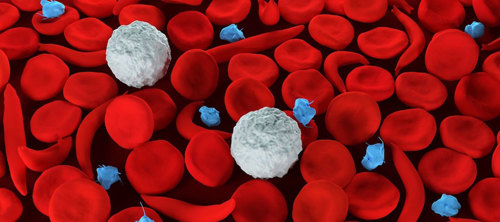
This is why the next step in making CRISPR ready for treating genetic diseases is to boost its editing efficiency. We want more cells to have the changed DNA from the very beginning.
And this isn’t the only part we want to improve either. It would also be nice if we could make it better at targeting. The CRISPR system is amazingly good at getting to the right spot in the DNA but it isn’t perfect. Sometimes it changes DNA where it shouldn’t.
These “off-target” effects can have serious consequences like causing a new genetic disease. Obviously we want to avoid that as much as possible!
So the CRISPR system is amazing enough to transform biology but not yet perfect. With a few improvements, it may just allow us to finally cure some deadly genetic diseases. And once these improvements are made, hopefully we will use this powerful new tool wisely.
Getting There
DNA is made up of four bases: A, G, C, and T. The instructions for you are written with a long string of 6 billion or so of these four bases.
CRISPR relies on something scientists Watson and Crick figured out back in 1953 — A always pairs with T and G with C. This underpins why a cell can easily copy its DNA when it gets ready to divide, how a cell reads its genes and lots of other aspects of how a cell works.
It is also how the CRISPR system works. There is a part of CRISPR called Cas9, which is the enzyme that actually cuts the DNA. Cas9 uses a close relative of DNA called RNA to get to the right spot.
RNA is very similar to DNA, though there are a few differences. While DNA has two strands (a double helix!), RNA only has one strand. And it doesn’t have any T’s. Instead, an A pairs up with a U.
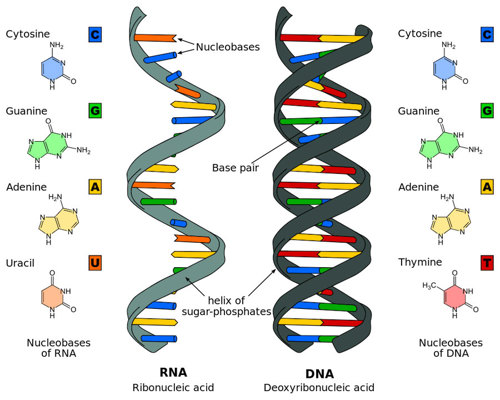
CRISPR-Cas9 uses RNA like a homing guide. Basically a scientist makes a piece of RNA (or gets the cell to do it for them) that matches a part of the DNA near where he or she wants to make a change.
If the DNA sequence is:
AGTCATTTTC CCGAAACTTT
Then the matching RNA would be:
UCAGUAAAAG GGCUUUGAAA
(You may have noticed that RNA has U's instead of T's. U's act the same way as T's and pair with A's.)
Here is how the DNA and the RNA line up:
This targeted DNA is long enough that most likely no other DNA sequence matches it in the cell. You’d need to make sure, but the odds are that there wouldn’t be two identical sequences.
So that is how you program Cas9 to go to where you want to edit the DNA in a cell. You simply supply it with the right piece of RNA and that piece of RNA guides it to the right spot. That’s where this kind of RNA gets its name — guide RNA.
Older gene editing systems (like TALENs or Zinc fingers) required scientists to redesign an enzyme for every new target. Turns out it is much harder to redesign an enzyme than it is to make a piece of RNA. Redesigning an enzyme is like writing a set of new code for your mapping app instead of just typing in a new address. This is why being able to use a guide RNA in CRISPR-Cas9 is so revolutionary!
Editing the DNA
Cas9, the enzyme part of the CRISPR system that gets the ball rolling on gene editing, is really just a nuclease. This is an enzyme that cuts DNA.
Cells HATE it when you cut their DNA because this can do all sorts of awful things to the cell.
Think about it like an electrical line that is downed in a storm. The end whips around dangerously, threatening people around it.
Now a cell won’t get electrocuted with the end of the DNA flailing around inside of it. But that naked end of DNA can cause problems with the rest of the cell’s DNA that can end up killing the cell just as surely as if it had been electrocuted.
This is why the cell immediately (and not very carefully) tries to patch the DNA break. Very often, to do this as quickly as possible, the cell adds a small amount of DNA or takes some away. It is like using duct tape to seal the ends of that electrical wire and then reconnecting them.
As you can imagine, that electrical wire doesn’t work anymore with duct tape blocking the current flow. And more often than not, the DNA doesn’t work either after being inexpertly stuck back together again.
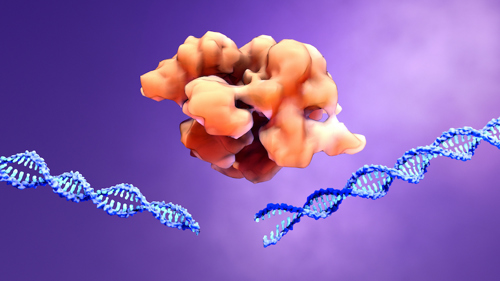
Remember, DNA is really a set of instructions. If you take away or add to it, those instructions can become garbled. It is like starting out with:
Add 2 tablespoons of sugar
And then going to:
Add 200 tablespoons of sugar
Or:
Add 2 spoons of sugar
In neither case are you going to end up with what you want! The same is true sometimes in our DNA.
If all Cas9 could do was damage the instructions in DNA, it would only be useful in a few special cases.
For example, people whose CCR5 genes don’t work are very resistant to HIV, meaning they are less likely to end up with AIDS than someone with a functional CCR5 gene. So wrecking someone’s CCR5 gene is probably a good thing and in fact is being used as a way to treat HIV right now.
But there aren’t a lot of cases like this. To be really useful, you want to be able to take a broken gene and fix it in an exact way. You want to take back the two zeroes in “Add 200 tablespoons of sugar” and get back to the original “Add 2 tablespoons of sugar.”
To do this scientists need to add in a piece of DNA that has the edit they want. The cut in the DNA activates a system in the cell that can then swap the new piece of DNA for the old, damaged one. The end result is an edited piece of DNA.
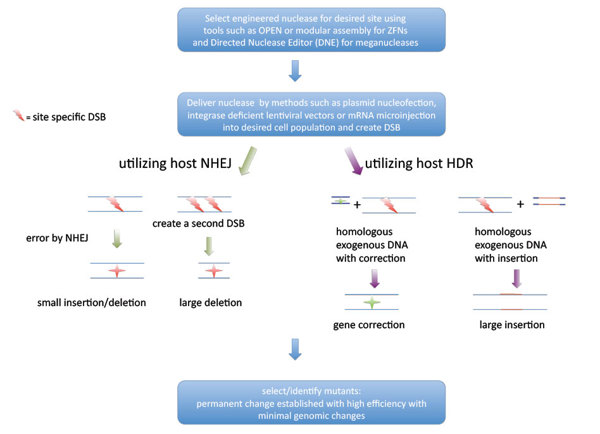
So Cas9 and the cell don’t really remove the two zeroes from “Add 200 tablespoons of sugar”. Instead, the cut by Cas9 essentially activates a system in the cell that cuts out the entire phrase “Add 200 tablespoons of sugar” and pastes “Add 2 tablespoons of sugar” in its place.
Getting More Efficient
If CRISPR could do this in most cells, we’d be able to cure a whole lot of genetic diseases. But it can’t.
It takes real effort to get 5% of cells to have their DNA changed in the right way. And when that happens, often many more cells have damaged DNA instead of edited DNA.
So the next steps are really to get more cells with changed DNA and fewer cells with damaged DNA.
As discussed here, a new study has tried to fix this by completely redesigning Cas9. It still gets to the right spot in the same way but once there, it does something completely different.

In essence, it changes the letters in the instructions instead of cutting the DNA. So if the instructions read “Add 2 cups sugar” instead of “Add 4 cups of sugar,” this new Cas9 will simply change the 2 to a 4 rather than pasting the old sentence over the new one.
And it will do it with a much higher efficiency. Enough cells can have the changed DNA that it might actually do some good.
It also damages the DNA much less often. One version, which doesn't cut the DNA, has hardly any damaged DNA.
Sounds great but unfortunately the new system isn’t as versatile as the old one. It can really only turn a C into a T or a G into an A. If the mutation causing your disease is one in which you have a C instead of a G, the new Cas9 won’t work. It can’t make this change.
With a few improvements, CRISPR just might revolutionize health care like it has already transformed biology research. Of course, we have heard this before with other techniques. We’ll need to wait and see if this one actually pans out.
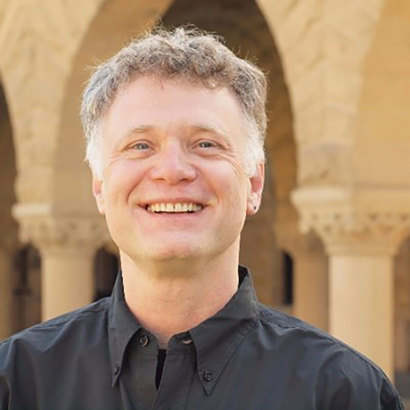
Author: Dr. D. Barry Starr
Barry served as The Tech Geneticist from 2002-2018. He founded Ask-a-Geneticist, answered thousands of questions submitted by people from all around the world, and oversaw and edited all articles published during his tenure. AAG is part of the Stanford at The Tech program, which brings Stanford scientists to The Tech to answer questions for this site, as well as to run science activities with visitors at The Tech Interactive in downtown San Jose.